
If you heat solid matter, it first melts and then vaporizes. So, what happens when gaseous matter is heated further and further? The heating gradually dissolves all the structures of matter and the molecules of matter are transformed into individual atoms. Eventually, when the temperature reaches around 1012 Kelvin (1,000,000,000,000°C), the components of the atomic nuclei, the protons and neutrons, are also melted and the fundamental building blocks, the quarks and gluons, emerge.
This high temperature, which is approximately one billion times higher than the interior of the sun, is where matter exists in its most elementary form imaginable today. This elementary state of matter is predicted by the standard model of particle physics and is referred to as quark-gluon plasma. This is a plasma of free quarks that interact with each other through the exchange of gluons. Our current understanding of the early universe indicates that our entire universe was filled with such a quark-gluon plasma in the first microseconds following the Big Bang. Lead atomic nuclei are brought to collision at high energy in the world's largest accelerator ring, the Large Hadron Collider (LHC), in order to investigate the properties of this plasma and in doing so, reconstruct the conditions of the early universe. The atomic nuclei are heated to the necessary temperature by the energy released during the collision and a quark-gluon plasma is generated for a brief period.
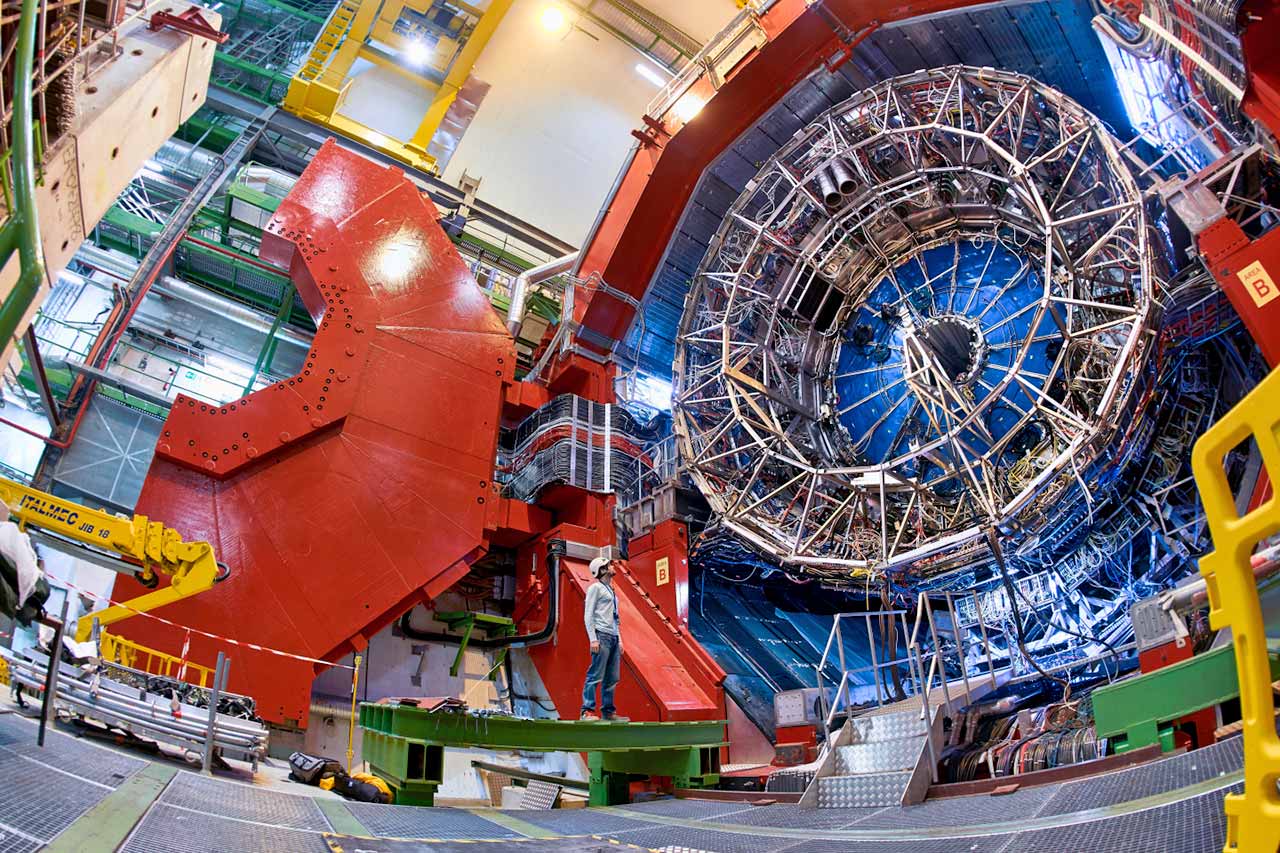
The Detector
Just like water vapor, whose cooling causes it to condense into small droplets, the quark-gluon plasma also freezes out after a short time. The resulting particles can be detected with the ALICE detector, one of the four large detectors at the LHC.
The unique challenge with ALICE lies in the fact that a single collision of two lead nuclei produces many thousands of particles, which need to be measured as comprehensively as possible. After all, it is only by precisely measuring as many particles and their properties as possible that physicists will succeed in gaining a more precise understanding of the processes taking place in the plasma. Several detector layers were built in a concentric manner around the collision point at the LHC in order to record the various properties such as momentum, energy and charge of the particles. These sub-detectors measure around 26 meters long and 16 meters wide and are situated inside a massive magnet weighing over 8,000 tons.
In comparison to other large-scale detectors, the ALICE experiment lends itself particularly well to determining the type of particle of almost all particles. This is possible thanks to the skillful use of a variety of physical processes such as the Cherenkov effect or transition radiation and the precise measurement of physical quantities such as time of flight and energy loss. As such, ALICE provides unique conditions for investigating the quark-gluon plasma.
Around 2000 scientists from over 40 countries are involved in setting up and operating the ALICE detector and assessing the data. Some 200 of these researchers come from Germany.
Current Research Issues
The formation of quark-gluon plasma at high temperatures was predicted as a fundamental property of matter as part of the standard model and is now regarded as experimentally confirmed. Hence, the current ALICE research program addresses further questions and investigates the detailed properties of the quark-gluon plasma. The viscosity of the plasma is being investigated, among other things, since this property possibly had an influence on the dynamics of the early universe. These and other measurements, such as the diffusion properties of quarks in the quark-gluon plasma or the order of the phase transition to normal nuclear matter, allow for detailed tests of some of the least understood aspects of the standard model. This involves the behavior of the strong interaction between quarks at distances where conventional mathematical methods no longer apply, but which is crucial for the appearance of our observable world, such as the formation of protons, neutrons and atomic nuclei.
Neutron Stars and the Search for Dark Matter
The question of whether quark-gluon plasma continues to exist in the interior of compact objects such as neutron stars can also be addressed with ALICE. The high sensitivity of the individual sub-detectors also facilitates unique studies of the properties of exotic particles and states of matter. The interaction between so-called strange particles or the formation of nuclear states consisting of more than just protons and neutrons, for example, can be investigated with ALICE. Such particles may also be found inside neutron stars and significantly shape their properties.
Another example of the broad research field of ALICE is how to determine the absorption properties of antimatter in intergalactic space. Precise knowledge of these properties plays a decisive role in the search for dark matter using extraterrestrial experiments.
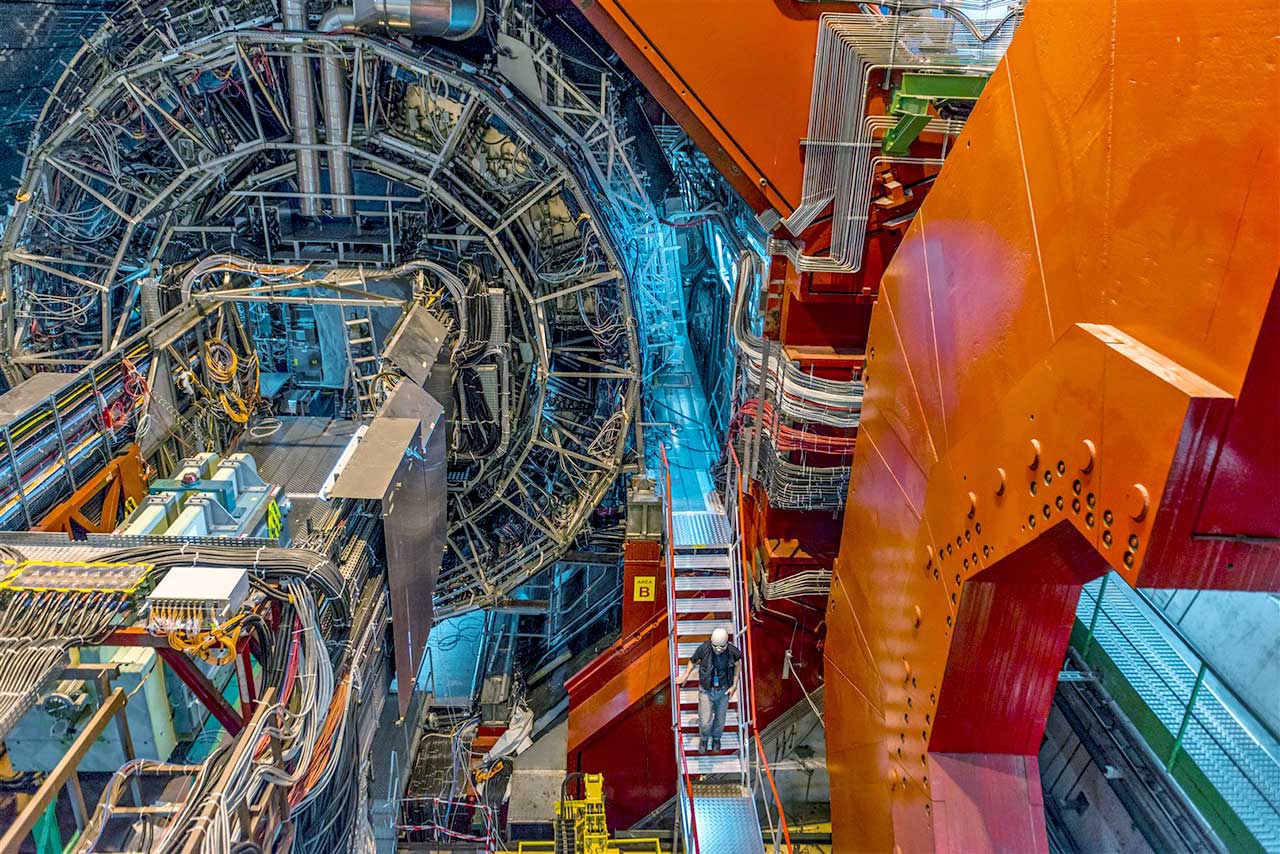
The ALICE Experiment – Profile
Dimensions:
- 16 m height, 16 m width, 26 m length
- > 8000 tons in weight
Location:
- St. Genis, France, 56 m underground
International Collaboration:
- 40 countries
- 127 institutes
- ~ 2000 scientists
German Involvement:
- 10 institutes
- > 200 scientists
- > 50 doctoral candidates
- > 100 completed doctorates
Impressions from the ALICE Collaboration
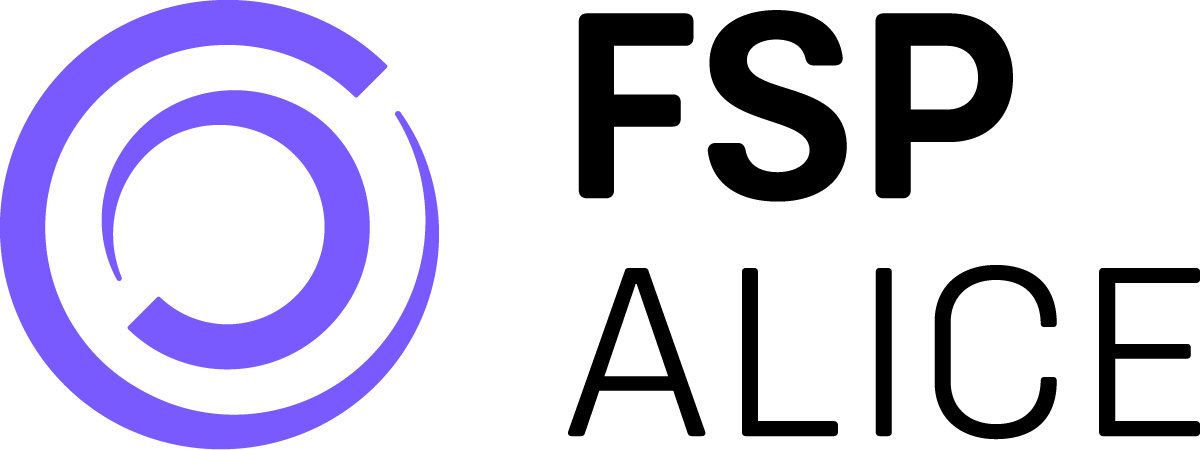
BMBF Research Priority Program ALICE
The work performed by German scientists in the ALICE collaboration receives significant funding from the German Federal Ministry of Education and Research (BMBF). Researchers from the universities of Bonn, Frankfurt, Heidelberg, Munich and Münster as well as the Helmholtz Centre for Heavy Ion Research GSI near Darmstadt are currently involved in ALICE. The BMBF has set up the ALICE Research Priority Program (FSP ALICE) to strengthen networking between the university groups and to promote joint measures to support young researchers, public relations work and the transfer of knowledge to society. The Technical University of Munich currently holds speakership of the FSP ALICE.
The German research groups play a role in all aspects of the ALICE experiment. They make a decisive contribution to the development and construction of the detector as well as to the operation and evaluation of the collected data. Outstanding expertise is demonstrated by the German groups, particularly in the development of track detectors and in the field of data readout and online computing. The physical interpretation of the results is supported by theory groups from Bielefeld, Münster and Tübingen.
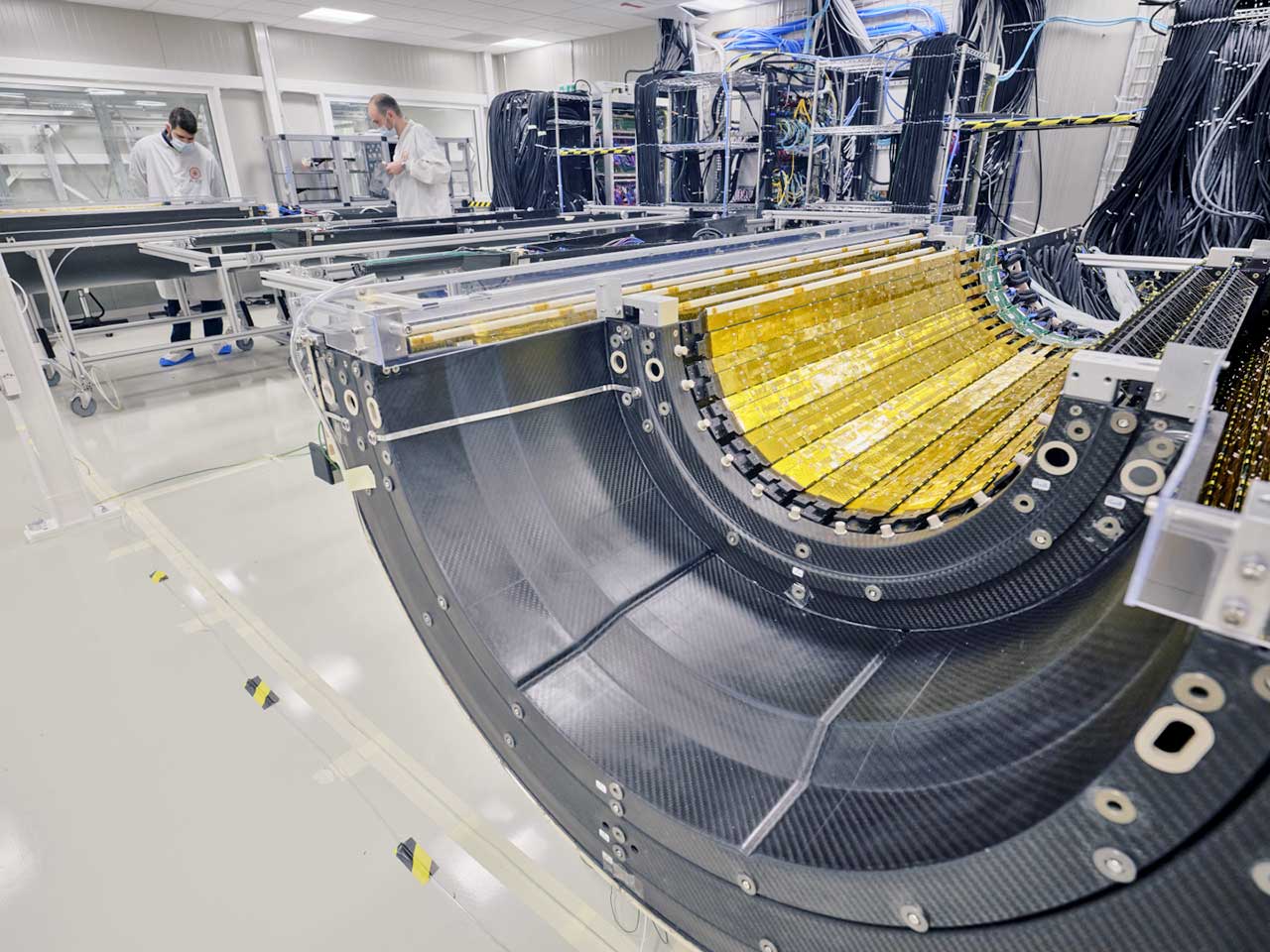
The Upgrade
In 2012, a decision was made to comprehensively upgrade the ALICE experiment with the aim of gaining an even more detailed understanding of the fundamental questions surrounding the quark-gluon plasma. Switching from event-based to continuous readout is expected to increase data recording a hundredfold. The scientists hope that a larger data set will provide them with more insights into the original state of our universe.
The German groups were heavily involved in this modernization, which received significant funding from the BMBF. The modernization was successfully completed in 2022, with the result that measurements at the LHC have continued since with significantly increased collision rates.
A particular challenge, in addition to the increased radiation resistance of the detector, which is necessary for smooth operation at high collision rates, is the immense amount of data that will be generated at ALICE following the upgrade. Data rates of approx. 4 terabytes per second require massive parallel processing in real time before the data is written to mass storage. To achieve this, complex large computer architectures were developed and constructed. Highly efficient GPU processors, which are also used in graphics cards, are used alongside conventional CPU processors. These computer structures represent a highly innovative approach and will also be used in other areas of science and industry.
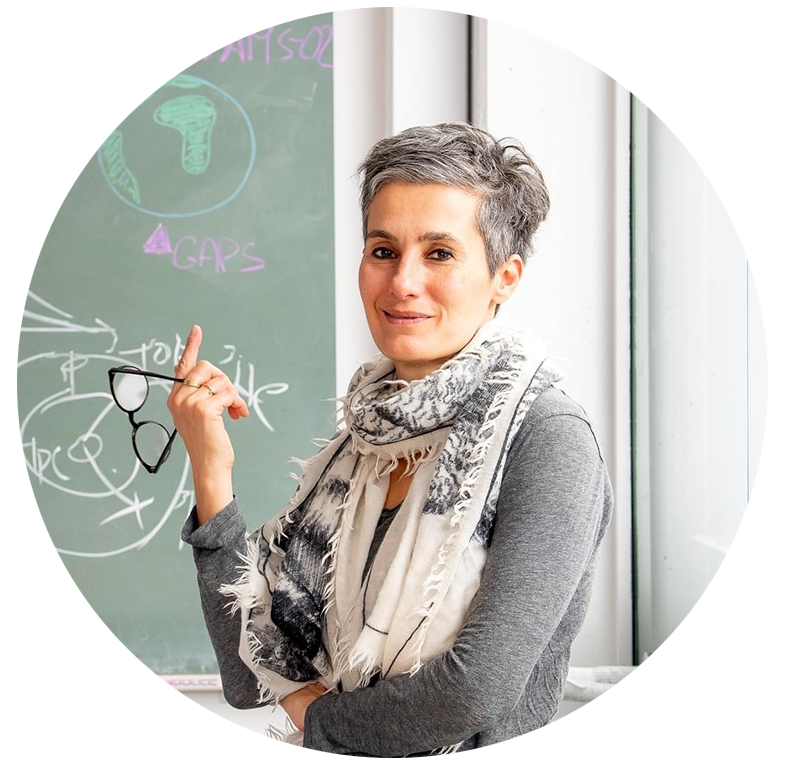
Spokesperson for the ALICE Research Priority Program
Prof. Dr. Laura Fabbietti
Faculty of Physics
Technical University of Munich (TUM)